Centre for Bioengineering
Research Themes
Predictive Modelling
- Predictive Bioengineering models provide faster, cheaper, and more reliable and ethically acceptable predictive testing.
- Organ-on-chip models mimic the physiological and pathological environment of different internal organs for drug testing.
- Computational and experimental testing methodologies predict the performance of biomaterials and medical devices.
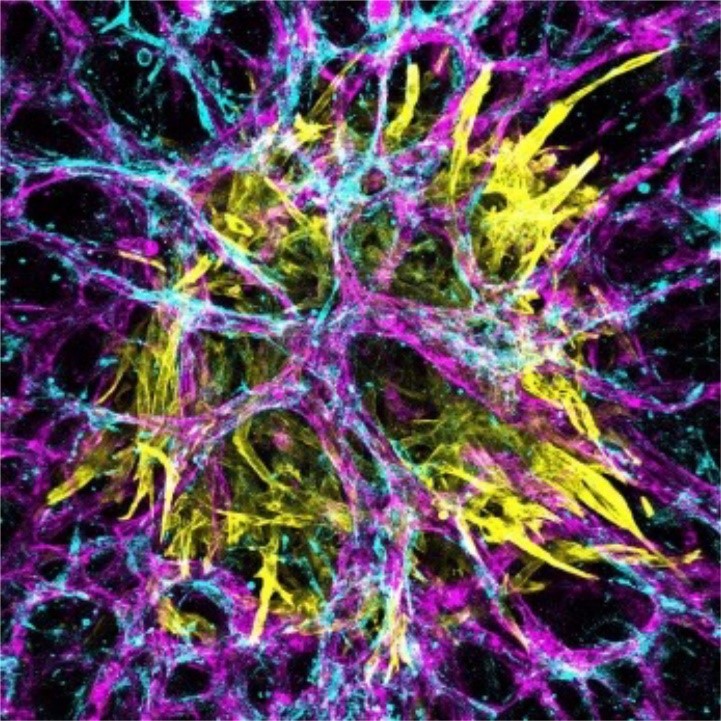
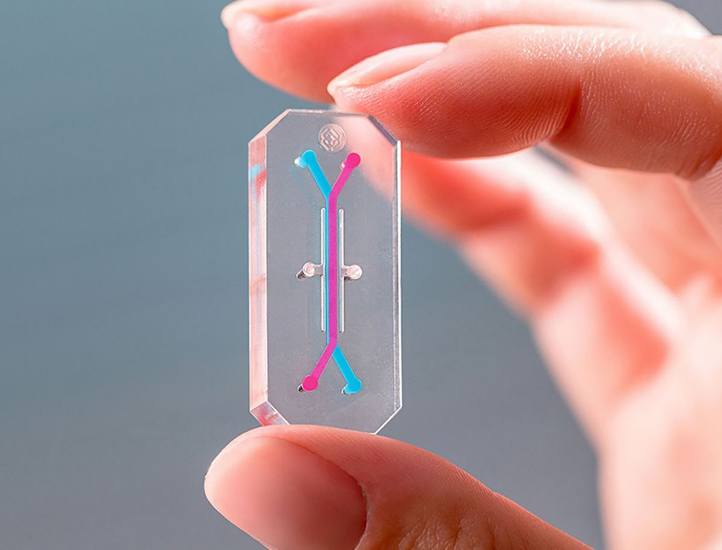
- Predictive Bioengineering is underpinned by multidisciplinary expertise in biomaterials, microfluidics, biomechanics, sensors, and computational modelling.
- Ongoing projects in organ-on-chip models and in vitro models for cancer, cartilage injury, fibrosis, orthopaedic biomaterial and cardiovascular device testing platforms.
Contact: Dr Thomas Iskratsch, Prof Hazel Screen, Prof Martin Knight
Biomaterials and Bio-interfaces
- Bioink design for 3D Printing and advanced in vitro models and organ-on-chip systems.
- Biosensor and smart surface design: Real-time optical and functional electro-chemical imaging at the cell and tissue level.
- Dynamic self-assembled systems for smart self-healing and environmentally responsive biomaterials and coating technologies.
- Micro and nanofabrication for life sciences.
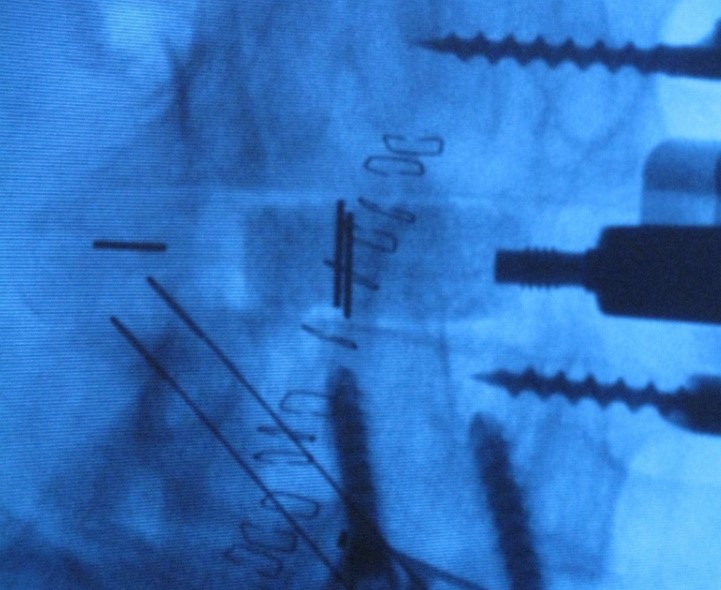
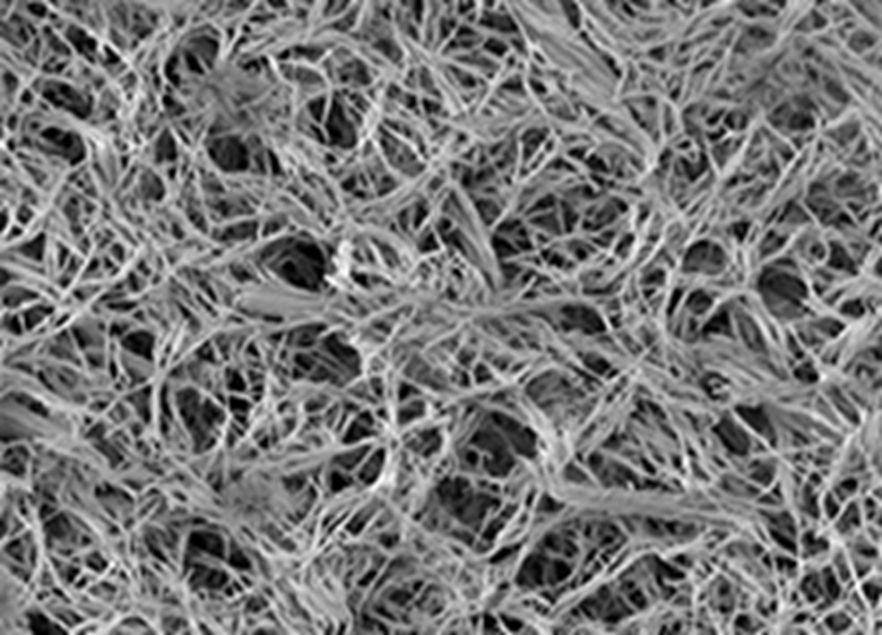
- Nanotherapeutics engineering: microcapsules, lipid nanoparticles and polymer nanoparticles for drug/gene delivery.
- Soft matter biomaterials engineering: Hydrogels, polymer brushes, emulsions for stem cell technologies, drug delivery and tissue engineering applications.
- Synthetic biology for biomaterials design.
Contact: Dr Karin Hing, Prof Gleb Sukhorukov, Prof Julia Shelton, Prof Julien Gautrot, Dr Stefaan Verbruggen
Biomechanics and Mechanobiology
- Mechanical and physical cues by cells in embryogenesis, bone formation and remodelling, and vascular remodelling after exercise.
- Disease related processes, such as metastasis, atherosclerosis, and hearing/pain sensing.
- Mechanical stimuli in health and disease response, looking at the whole-body down to individual molecules.
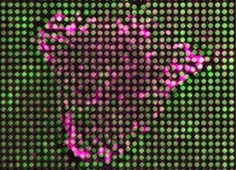
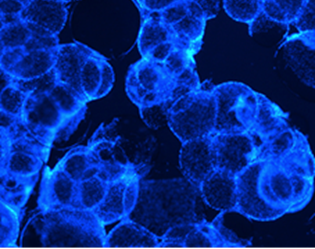
- Biophysical molecular processes regulating mechanotransduction, and the dynamical (epi)genetic responses of mechanotransductive pathways.
- Translational bioengineering to develop novel implantable biomaterials, devices, and tissue engineered products.
- Biological response of cells and tissues to biomechanical, topographical, and physiochemical stimuli in health and disease.
Contact: Dr Karin Hing, Prof David Lee, Prof Hazel Screen, Prof Julia Shelton, Prof Martin Knight, Prof Rob Krams, Dr Tina Chowdhury, Dr Stefaan Verbruggen
Bioprinting, Biofluids, and Regenerative Medicine
- 3D printing of bacterial communities for form and function testing.
- State-of-art droplet-based and light-based additive bioprinting technologies and predictive multi-scale in vitro models of human tissue.
- 3D printing of microrobots, drug delivery vehicles and soft actuation mechanisms.
- Biofluid mechanics for modelling, simulation and characterisation of flowing biological cells.
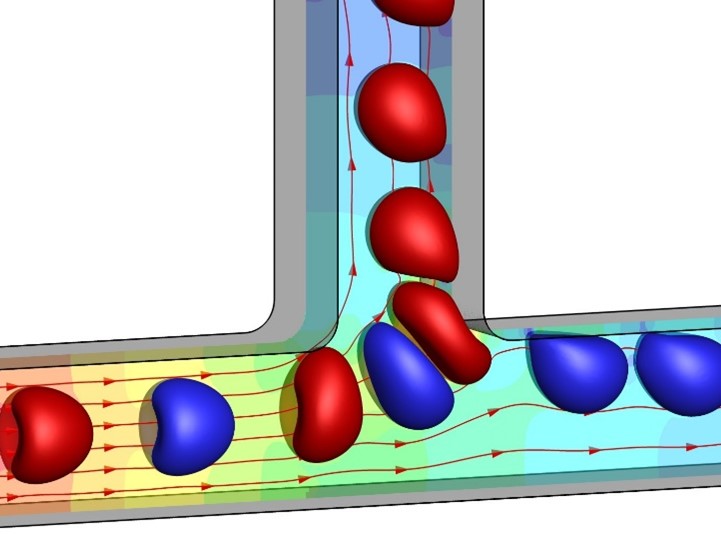
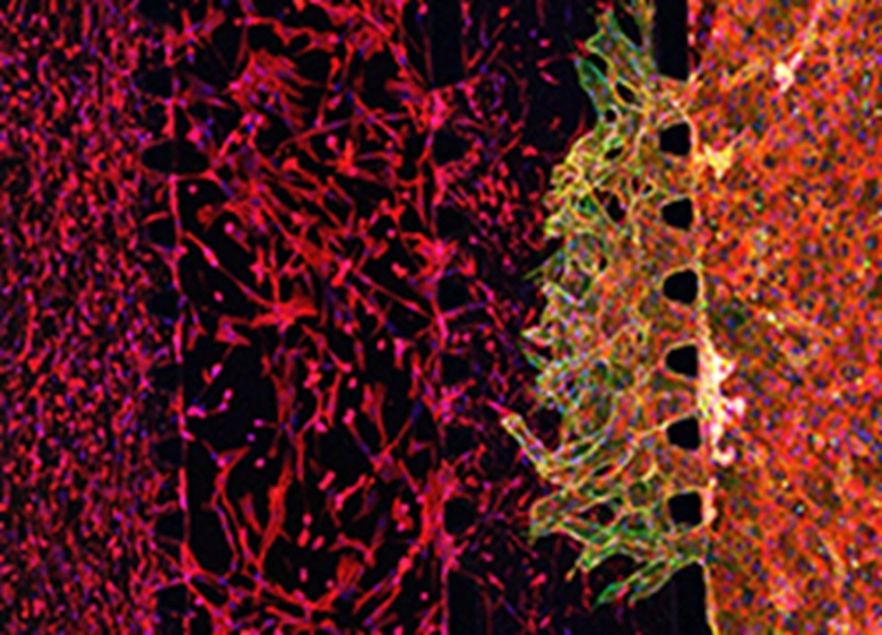
- Using light-based additive manufacturing processes to produce 3D polymeric objects volumetrically and all-at-once through tomographic photopolymerization.
- Developing functional human tissue and organ models using advanced 3D printing techniques.
- Discovering new and advanced photo-active biomaterials and links.
- Molecular techniques are used to create a high throughput CRISPR validation platform for drug studies.
- This CRISPR platform is used in the evaluation of all the interactions within a cell (interactome).
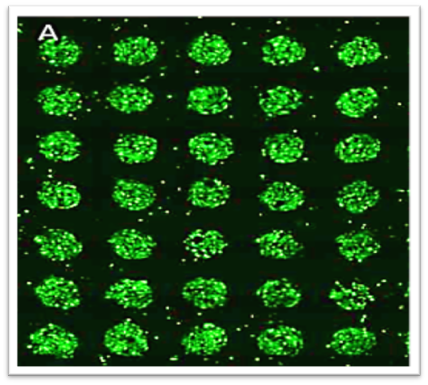
Contact: Prof David Lee, Dr Hossein Heidari, Prof Rob Krams, Prof Julia Shelton, Dr Tina Chowdhury, Prof Wen Wang, Prof Yi Sui, Dr Ravinash Kumar
Healthcare Robotics and MedTech
- Digital health in the form of smart wearable technology and mobile health.
- The human-computer interaction, where the user or patient is considered as part of the hardware loop.
- Combining medical images with artificial intelligence to help with diagnosis and treatment.
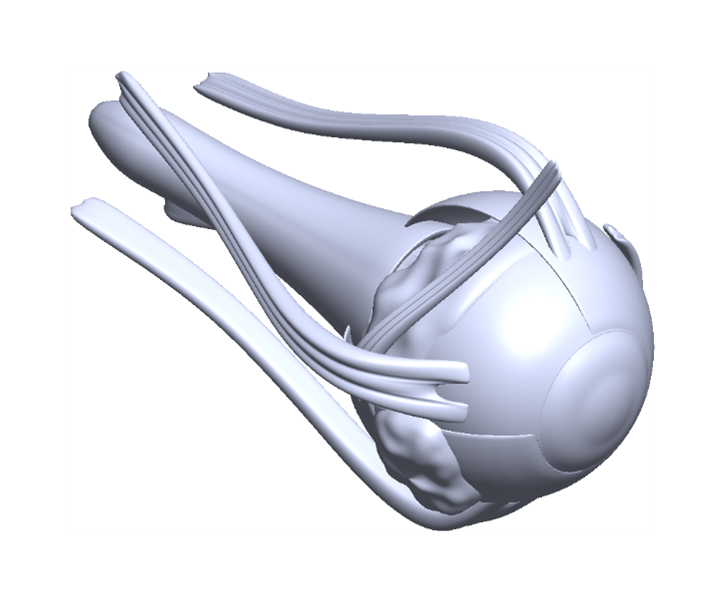
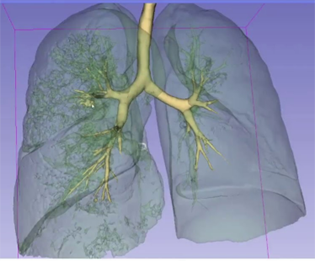
- Medical mechatronics, surgical robotics, and medical imaging to soft robotics and biorobotics.
- Our broad scope of topics lends itself to multidisciplinary translational research.
Contact: Prof Zion Tse, Dr Caroline Roney, Dr Himadri Gupta, Dr Lei Su, Dr Mohammad Hassannezhad, Dr Thilina Lalitharatne, Dr Ildar Farkhatdinov
AI and Data Modelling for Health and Wellbeing
- Deep learning approaches for large imaging datasets: segmentation, identifying, biomarkers, diagnosis and prognosis.
- Signal processing of cardiac arrythmia data.
- Collaborating on developing an ecosystem for digital twins in healthcare.
- Development of personalised cardiac models and virtual cohorts for in silico trials.
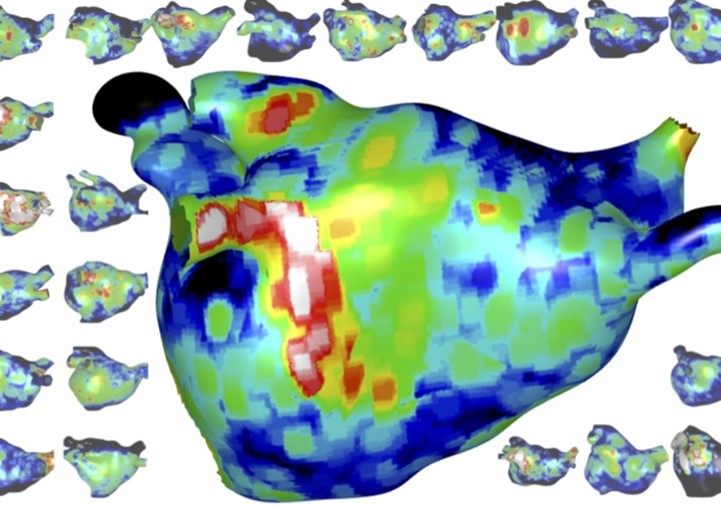
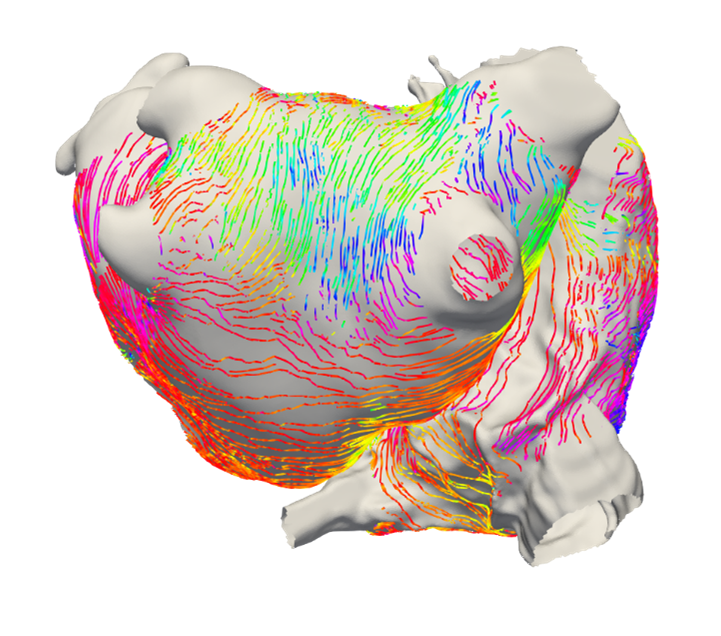
- Combining biophysical simulation and machine learning to predict patient trajectories, e.g. response to catheter ablation therapy using virtual patient cohorts.
- Machine learning models to predict the risk of preterm birth.
- Combining biophysical simulation and machine learning to speed up fluid mechanics simulations.
- Prediction of optimal patient-specific treatment strategies.
- A physics-based artificial intelligence approach for use in digital twins is used to analyse shear stress and wall stress on both cells in a culture and animal cells, returning results within minutes.
- A combination of engineering techniques and molecular techniques are used to study and understand the interaction of gene expression.
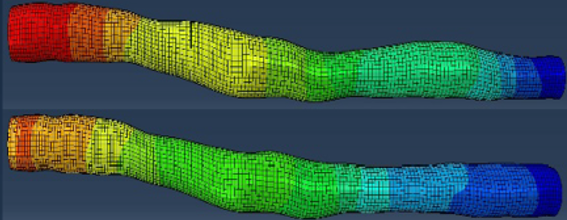
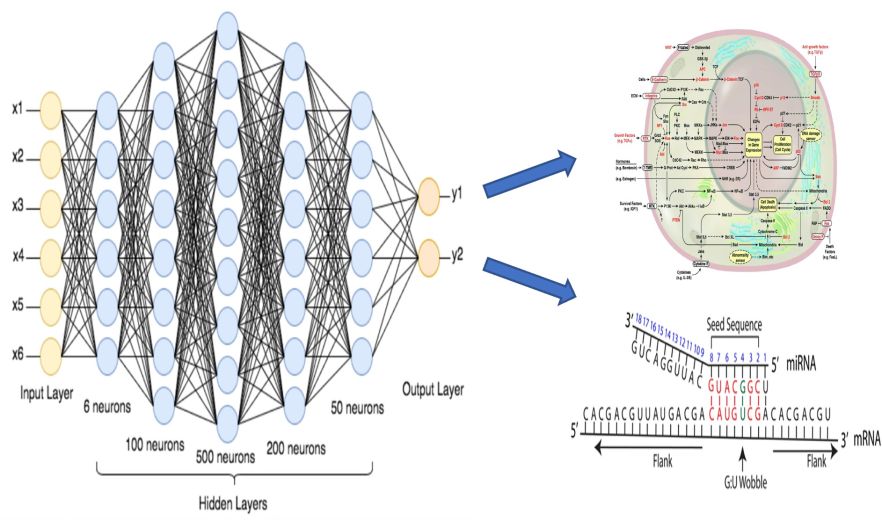
- Molecular techniques are combined with artificial intelligence to aid in the detection of novel RNA drugs.
Contact: Dr Caroline Roney, Prof Rob Krams, Prof Wen Wang, Prof Yi Sui, Dr Thilina Lalitharatne, Dr Ildar Farkhatdinov, Prof Zion Tse
Sensing and Imaging
- Optical-fibre imaging technology enabled ultrathin and high-resolution micro-endoscopes
- Wearable optical and ultrasound sensors for preclinical testing or next-generation homecare devices
- Machine learning and artificial intelligence assisted sensing and imaging in healthcare.
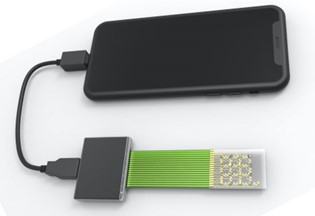
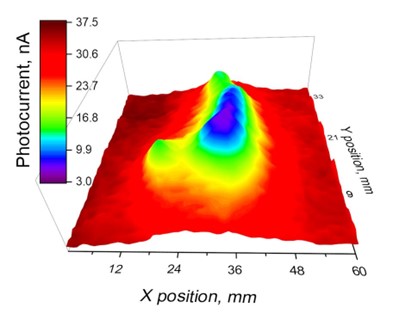
- Photoelectrochemical imaging (PEI) for monitoring cell signaling and cell metabolism with micron resolution.
- A combination of PEI and scanning ion conductance microscopy (SICM) allows functional and topological imaging of cells on basal and apical sides simultaneously.
- 3D photoelectrochemical imaging is being developed for the investigation of cell responses in 3D tissue culture and mapping photocatalytic activity in porous semiconductors.
Contact: Prof Zion Tse, Dr Himadri Gupta, Dr Lei Su, Prof Rob Krams, Prof Steffi Krause, Dr Thilina Lalitharatne, Dr Ildar Farkhatdinov
Diversity of Ideas
We believe that a diversity of ideas helps us achieve the previously unthinkable. Throughout our history, we’ve improved lives through academic excellence, and we continue to live and breathe this spirit today. Our reformer heritage informs our conviction that great ideas can and should come from anywhere. It’s an approach that has brought results across the globe. We continue to embrace diversity of thought and opinion in everything we do, in the belief that when views collide, disciplines interact, and perspectives intersect, truly original thought takes form.